Video Gallerie
» Large Eddy Simulation (LES) turbulenter Verbrennung
- Turbulente Strömung und Flammenausbreitung durch 8 rotierende Ventilatoren innerhalb des Explosionsbehälters unter erhöhten Druckbedingungen
Geometry and computational mesh with 8 rotating fans and local refinement |
Grid of the ventilator surface and a slice across the fan
LES of turbulent flow generated by rotating ventilators
Contours of velocity and vorticity on a slice across the rotating fan.
Velocity (top) and vorticity (bottom) fields calculated by LES with moving fans at different pressure conditions: 1 bar (left), 2 bar (middle) and 5 bar (right). Increasing pressure leads to generation of smaller turbulence structures.
LES of ignition process of a methane/air mixture at ϕ = 0.9 and T0 = 300 K under turbulent flow conditions generated by rotating fans (5000 rpm) within the bomb vessel at different pressures: 1 bar (left) and 2 bar (right)
Time evolution of 3D flame surfaces (top) and 2D contours of temperature (bottom) by LES of ignition of a methane/air mixture at 1 bar (left), 2 bar (middle) and 5 bar (right).
- Lean-premixed, highly turbulent natural gas/air combustion at preheated condition (Matrix Flame)
Overall simulation illustrated by contours of temperature and axial flow velocity : non-reactive flow → ignition → flame propagation → Extinction
Contours of axial velocity and vorticity illustrating strong turbulent inflow generated at the inlet boundary and its interaction with the flame front
Effect of the inflow turbulence on the flame length: LES without (left) and with (right) prescribed turbulence at inlet |
Streamwise velocity used at the inlet boundary |
- Partially premixed methane/air combustion in a vitiated coflow (Cabra Flame)
Overall simulation : mixing of cold main jet with hot coflow → ignition → Stabilization at lift-off height. From left to right are contours of streamwise velocity, mixture fraction, temperature, mass fraction of OH and reaction progress variable
- Non-premixed H2/air combustion (Sandia H3 Flame)
Flame surface is illustrated by the iso-contour of stoichiometric mixture fraction; horizontal line indicates measured length of flame
-
Magere vorgemischte Erdgas-Luft-Verbrennung, stabilisiert durch einen doppelt konzentrischen Drallbrenner (GCN)
- Bender, C. and Büchner, H. (2005): Noise emissions from a premixed swirl combustor, Twelfth International Congress on Sound and Vibration, Lisbon.
- Habisreuther, P., Bender, C., Petsch, O., Büchner, H. and Bockhorn, H. (2006): Prediction of pressure oscillations in a premixed swirl combustor flow and comparison to measurements, Flow Turb. Comb.77, 147-160.
Swirl-stabilized combustion with a recessed pilot lance & without confinement. From left to right and top to bottom are contours of streamwise velocity, vorticity, temperature and progress variable. The inner recirculation zone moves back and forth into the burner
Isoterm of T = 1500 K and iso-contour of u = - 1 m/s
Swirl-stabilized combustion with a planar pilot lance and a combustion chamber, other boundary conditions are remained the same
-
DTBP Auftriebsflamme in Einfach- und Mehrfachanordnung (Poolflamme)
Development of a DTBP buoyant flame in single pool arrangement: from left to right are contours of streamwise velocity, mixture fraction, temperature and reaction progress variable
Iso-surface of reaction rate and temperature
DTBP pool flame in multiple arrangement. Merging of single pool flames to a large fire
-
Vorgemischte Erdgas-/Luftstrahlflamme, die mit einem generischen Brenner betrieben wird
Moderately turbulent partially-premixed flame with generation of large coherent flow structures which interact with the flame: from left to right are contours of temperature, heat release rate, reaction rate and mass fraction of chemiluminescent OH∗ species
Isoterm of T = 500 K: evolution of the flame with the coherent vortices leads to formation of a hazelnut-shaped structure of the flame surface
» Direkte numerische Simulation (DNS) der Verbrennung
-
Ungespannte planare vorgemischte Flammen (1D)
Freely propagating methane/air flame: ϕ = 1.0, T0 = 300 K, p0 = 1 atm. The flame is ignited by a heating source within the domain. The result has shown a good agreement with the computational data obtained with the commercial code Chemkin.
-
Instationäre/oszillierende gestreckte Flammen (2D)
Harmonically excited plane-jet hydrogen/air flames with different frequencies and the same amplitude: ϕ = 0.5, unstable region with Le<1, Ma<0. The flame responds faster to the unsteady flow at low frequencies and tends to form instabilities at higher frequencies. At very high frequencies the flame's response is attenuated again.
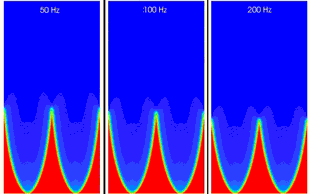
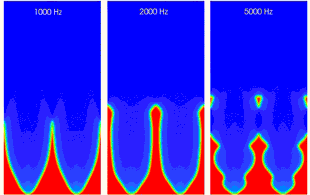
Harmonically excited hydrogen/air flames with different frequencies and the same amplitude: ϕ = 0.8, neutral range with Le ≈ 1, Ma ≈ 0.
Harmonically excited hydrogen/air flames with different frequencies and the same amplitude: ϕ = 6.5, stable range with Le > 1, Ma > 0.
-
Sphärische Flammenausbreitung in einem geschlossenen Bombenschiff (3D)
DNS of spherically expanding H2/air flame at ϕ = 0.4 fora 3D grid with 144 million grid cells, the flame surface becomes unstable at large flame radii due to thermo-diffusive instability (Le<1). The DNS has been run in parallel with 8192 CPU cores on the Cray-XC40 platform from HLRS.
Isoterm of T = 1100 K showing collapse of the spherical flame surface into unstable, cellular structure.
Vector-plots of velocity field generated by the spherically expanding flame.
Contours of heat release rate: DNS of spherical propagating flame with a dynamically refined mesh.
-
Synthetische Methan-/Luftflammen bei unterschiedlichen Turbulenzbedingungen (3D)
Synthetical flame front subjected to a turbulent inflow. Case I: the flame is convected by the flow to the outside of the computational domain.
Interaction of turbulent flow with flame. The flame front is illustrated with isotherm of T = 1500 K and structures of the vortices are depicted by iso-surfaces of the vorticity.
Case II: the inflow conditions are adjusted to let the flame propagate within the computational domain. From left to right are cases with different turbulent Reynolds numbers of Ret = 15, 69 and 123. The cubic domain of 1 cm3 has been discretized equidistantly with 64 million finite volumes. The used reaction mechanism for methane/air combustion contains 19 species and 69 elementary reactions.
-
DNS eines generischen Brennersystems (3D)
Contours of resolved temperature and heat release rate (left). Comparison of contours of heat release rate and OH* mass fraction (right).
|
Zoomed flame tip region showing resolution of the flame front by the DNS |
|
» Acoustic modeling
-
Komprimierbares DNS des Trompetenflusses
Temporal evolution of acoustic pressure generated in the trumpet (till 1 m).
-
Verbrennungsinstabilitäten durch Wirbelbrenner
|
Combustion instabilities caused by Helmholtz resonators, standing waves and vortex shedding. |
|
Oscillation of temperature and flow fields caused by combustion instability. |
|
Combustion instability caused by coherent flow structure (left) and helical structures visualized by iso-surface of pressure (right). |
Aero-acoustic (left) and combustion generated (right) noise sources derived from Lighthill's acoustic analogy.
Acoustic sources given by the time derivative of the density.
-
Direktes Verbrennungsgeräusch
|
Propagation of acoustic waves from a turbulent premixed flame (compressible LES). The wave fronts are indicated by isocontours of the pressure. |
|
Reflection of pressure waves at the opening boundaries in the case of using a small computational domain. |
Acoustic noise sources derived from compressible LES of turbulent premixed combustion: aero-acoustic (left) and combustion (middle) noise sources obtained from Lighthilll's analogy and acoustic source caused by time fluctuation of heat release (right).
Publikationen
2020
-
Galeazzo, Flavio Cesar Cunha; Zhang, Feichi; Zirwes, Thorsten; Habisreuther, Peter; Bockhorn, Henning; Zarzalis, Nikolaos; Trimis, Dimosthenis; (2020). Vortrag: Implementation of an Efficient Synthetic Inflow Turbulence-Generator in the Open-Source Code OpenFOAM for 3D LES/DNS Applications. 23rd Results and Review Workshop of the HLRS, online, Oct. 08.-09.,
-
Hansinger, Maximilian; Zirwes, Thorsten; Zips, Julian; Pfitzner, Michael; Zhang, Feichi; Habisreuther, Peter; Bockhorn, Henning, (2020). The Eulerian Stochastic Fields method applied to Large Eddy Simulations of a piloted flame with inhomogeneous inlet. Flow, Turbulence and Combustion, 105, 837–867.(doi:10.1007/s10494-020-00159-5)
-
Wang, Yiqing; Zhang, Huangwei; Zirwes, Thorsten; Zhang, Feichi, Bockhorn, Henning; Zheng, Chen, (2020). Ignition of dimethyl ether/air mixtures by hot particles: Impact of low temperature chemical reactions. Proceedings of the Combustion Institute, in press.(doi:10.1016/j.proci.2020.06.254)
-
Zhang, Feichi; Zirwes, Thorsten; Häber, Thomas; Bockhorn, Henning; Trimis, Dimosthenis; Suntz, Rainer, (2020). Near Wall Dynamics of Premixed Flames. Proceedings of the Combustion Institute, in press.(doi:10.1016/j.proci.2020.06.058)
-
Zhang, Feichi; Zirwes, Thorsten; Habisreuther, Peter; Zarzalis, Nikolaos; Bockhorn, Henning; Trimis, Dimosthenis, (2020). Numerical Simulations of Turbulent Flame Propagation in a Fan-Stirred Combustion Bomb and Bunsen-Burner at Elevated Pressure. Flow, Turbulence and Combustion, online.(doi:10.1007/s10494-020-00209-y)
-
Zhang, Feichi; Heidarifatasmi Hossein; Harth, Stefan; Zirwes, Thorsten; Wang, Robert; Fedoryk, Michal; Sebbar, Nadia; Habisreuther, Peter; Trimis, Dimosthenis; Bockhorn, Henning, (2020). Numerical evaluation of a novel double-concentric swirl burner for sulfur combustion. Renewable and Sustainable Energy Reviews, 133, 110257.(doi:10.1016/j.rser.2020.110257)
-
Zirwes, Thorsten; Zhang, Feichi; Habisreuther, Peter; Bockhorn, Henning; Trimis, Dimosthenis, (2020). Quasi-DNS Dataset of a Piloted Flame with Inhomogeneous Inlet Conditions. Flow, Turbulence and Combustion, 104, (4), 997-1027.(doi:10.1007/s10494-019-00081-5)
-
Zirwes, Thorsten; Zhang, Feichi; Wang, Yiqing; Habisreuther, Peter; Denev, Jordan A.; Chen, Zheng; Bockhorn, Henning; Trimis, Dimosthenis, (2020). In-situ flame particle tracking based on barycentric coordinates for studying local flame dynamics in pulsating Bunsen flames. Proceedings of the Combustion Institute, 38, (online), (doi:10.1016/j.proci.2020.07.033)
-
Zirwes, Thorsten; Zhang, Feichi; Denev, Jordan A.; Habisreuther, Peter; Bockhorn, Henning; Trimis, Dimosthenis, (2020). Lagrangian Tracking of Material Surfaces in reacting Flows, in The 15th OpenFOAM Workshop, Washington, DC, USA, 22-25 June 2020, .
-
Zirwes, Thorsten; Häber, Thomas; Zhang, Feichi; Kosaka, Hidemasa; Dreizler, Andreas; Steinhausen,; Matthias Hasse, Christian; Stagni, Alessandro; Trimis, Dimosthenis; Suntz, Rainer; Bockhorn, Henning, (2020). Numerical Study of Quenching Distances for Side‑Wall Quenching Using Detailed Diffusion and Chemistry. Flow, Turbulence and Combustion, (online), (doi:10.1007/s10494-020-00215-0)
-
Zirwes, Thorsten; Zhang, Feichi; Denev, Jordan A.; Habisreuther, Peter; Bockhorn, Henning; Trimis, Dimosthenis; Poster: Implementation of Lagrangian Surface Tracking for High Performance Computing. 23rd Results and Review Workshop of the HLRS, online, Oct. 08.-09., 007-Rea-DNSbomb, 2020.
-
Zirwes, Thorsten; Zhang, Feichi; Habisreuther, Peter; Hansinger, Maximilian; Bockhorn, Henning; Pfitzner, Michael; Trimis, Dimosthenis, (2020). Identification of Flame Regimes in Partially Premixed Combustion from a Quasi-DNS Dataset. Flow, Turbulence and Combustion, (online), (doi:10.1007/s10494-020-00228-9)
2019
-
Denev, J. A.; Naydenova, I.; Zhang, F.; Zirwes, T.; Bockhorn, H., (2019). Unsteady pure straining effects on lean premixed flames of different Lewis numbers, in Proceedings of the European Combustion Meeting – 2019, April 14-17, Lisboa, Portugal, p. S4_AIII_37, .
-
Pausch, K; Sohel Herff, S.; Zhang, F.; Bockhorn, H.; Schröder, W., (2019). Noise Sources of Lean Premixed Flames. Flow, Turbulence and Combustion, 103, (3), 773-796.(doi:10.1007/s10494-019-00032-0)
-
Pausch, K.; Herff, S.; Zhang, F.; Bockhorn, H.; Schröder, W.; Poster: Analysis of Thermoacoustic Sources of Lean Premixed Flames. International Workshop on Clean Combustion: Principles and Applications, September, 25th-26th, Darmstadt, Germany, 2019.
-
Sebbar, N.; Harth, S.; Fedoryk, M.; Heidarifatasmi, H.; Zhang, F.; Bozzelli, J. W.; Bockhorn, H.; Trimis, D.; (2019). Vortrag: Sulfur combustion as closing step in a sulfur based solar-thermal cycle. COST Action SMARTCATS – 1st international conference on smart energy carriers, January 21-23, Napoli, Italy, invited lecture.
-
Zhang, F.; Heidarifatasmi, H.; Zirwes, T.; Fedoryk, M.; Harth, S.; Sebbar, N.; Habisreuther, P.; Trimis, D.; Bockhorn, H., (2019). Numerical simulation of sulfur combustors with high-power-density, in Proceedings of the European Combustion Meeting – 2019, April 14-17, Lisboa, Portugal, p. S2_AIII_57, .
-
Zhang, F.; Zirwes, T.; Habisreuther, P.; Bockhorn, H.; Trimis, D.; (2019). Vortrag: Spectral response of heat release in LES combustion modeling. Seventeenth International Conference on Numerical Combustion, 06-08 May, Aachen, Germany, MS8-208.
-
Zhang, F.; Zirwes, T.; Habisreuther, P.; Bockhorn, H.; Trimis, D.; (2019). Vortrag: LES of combustion noise from a turbulent premixed jet flame. Seventeenth International Conference on Numerical Combustion, 06-08 May, Aachen, Germany, MS8-210.
-
Zhang, F.; Zirwes, T.; Habisreuther, P.; Zarzalis, N.; Bockhorn, H.; Trimis, D., (2019). Numerical Simulation of Turbulent Flame Propagation in a Fan-Stirred Combustion Bomb at Elevated Pressures, in 27th International Colloquium on Detonation, Explosion and Reactive Systems (ICDERS), Juli 28.- August 2., Beijing, China, p. 1-6, .
-
Zhang, Feichi; Zirwes, Thorsten; Habisreuther, Peter; Zarzalis, Nikolaos; Bockhorn, Henning; Trimis, Dimosthenis, (2019). Numerical Computation of Turbulent Flows in a Fan-stirred Combustion Bomb. Combustion, Science and Technology, (online), (doi:10.1080/00102202.2019.1665520)
-
Zhang, Feichi; Hosein, Heidarifatasmi; Harth, Stefan; Zirwes, Thorsten; Sebbar, Nadia; Fedoryk, Michal; Habisreuther, Peter; Trimis, Dimosthenis; Bockhorn, Henning, (2019). Numerical Investigation of a Sulfur Combustor, in 29. Deutscher Flammentag, Deutsche Sektion des Combustion Institutes und DVV/VDI-Gesellschaft Energie und Umwelt, September, 17-18, Bochum., .
-
Zirwes, T.; Zhang, F.; Häber, T.; Bockhorn, H., (2019). Ignition of combustible mixtures by hot particles at varying relative speeds. Combustion, Science and Technology, 191, (1), 178-195.(doi:10.1080/00102202.2018.1435530)
-
Zirwes, Thorsten; Zhang, Feichi; Habisreuther, Peter; Bockhorn, Henning; Trimis, Dimosthenis, (2019). Large-Scale Quasi-DNS of Mixed-Mode Turbulent Combustion, in Book of Abstracts of the 90th Annual Meeting of the International Association of Applied Mathematics and Mechanics (GAMM2019), vol. 90, Vienna, Austria, February 18-20, p. 325, (ISBN 978-3-903024-84-7), .
-
Zirwes, T.; Häber, T.; Zhang, F.; Kosaka, H.; Bockhorn, H.; Suntz, R.; Dreizler, A.; Janicka, J., (2019). 2D and 3D numerical simulation of chemiluminescent radical concentrations during side-wall quenching of premixed methane and propane flames, in Proceedings of the European Combustion Meeting – 2019, April 14-17, Lisboa, Portugal, p. S3_AIII_47, .
-
Zirwes, T.; Zhang, F.; Habisreuther, P.; Denev, J. A.; Bockhorn, H.; Trimis, D.; (2019). Vortrag: HPC Impementation of Flame Particle Tracking for Studying Laminar and Turbulent Flame Dynamics. Seventeenth International Conference on Numerical Combustion, 06-08 May, Aachen, Germany, 242.
-
Zirwes, T.; Zhang, F.; Denev, J. A.; Habisreuther, P.; Bockhorn, H.; Trimis, D.; Improved Vectorization for Efficient Chemistry Computations in OpenFOAM for Large Scale Combustion Simulations. In High Performance Computing in Science and Engineering ’18, Nagel W., Kröner D., Resch M. (ed.), Springer, Cham, p. 209-224, (doi:10.1007/978-3-030-13325-2_13) 2019.
-
Zirwes, T.; Sebbar, N.; Habisreuther, P.; Harth, S.; Zhang, F.; Bockhorn, H.; Trimis, D., (2019). Ignition Behaviour of Sulfur in Air Based on Modified Reaction Kinetics, in Proceeding of the Eleventh Mediterranean Combustion Symposium - MCS11, June, 16-20, Tenerife, Spain, .
-
Zirwes, Thorsten; Zhang, Feichi; Habisreuther, Peter; Bockhorn, Henning; Trimis, Dimosthenis, (2019). Large-Scale Quasi-DNS of Mixed-Mode Turbulent Combustion. Proceedings in Applied Mathematics & Mechanics, 19, (1), (doi:10.1002/pamm.201900420)
-
Zirwes, T.; Häber, T.; Zhang, F.; Steinhausen, M.; Kosaka, H.; Bockhorn, H.; Stuntz, R.; Hasse, C.; Dreizler, A.; Poster: Numerical and Experimental Investigation of Chemiluminescent Radical Concentrations during Side-Wall Quenching. International Workshop on Clean Combustion: Principles and Applications, September, 25th-26th, Darmstadt, Germany, 2019.
-
Zirwes, T.; Zhang, F.; Habisreuther, P.; Hansinger, M.; Bockhorn, H.; Pfitzner, M.; Trimis, D.; Poster: Identification of Flame Regimes in Partially Premixed Combustion from a Quasi-DNS Dataset. International Workshop on Clean Combustion: Principles and Applications, September, 25th-26th, Darmstadt, Germany, 2019.
-
Zirwes, T.; Zhang, F.; Denev, J. A.; Habisreuther, P.; Bockhorn, H.; Trimis, D.; Enhancing OpenFOAM’s Performance on HPC Systems. In High Performance Computing in Science and Engineering '19, Nagel, W.E.; Kröner, D.H.; Resch, M.M. (ed.), Springer, p. accepted, 2019.
-
Zirwes, T.; Zhang, F.; Habisreuter, P.; Bockhorn, H.; Trimis, D.; (2019). Vortrag: Effect of Transient Flame Stretch. Simulation of reactive Thermo-Fluid Systems (STFS). Technical University Darmstadt. Germany, 22. September,
-
Zirwes, T.; Zhang, F.; Habisreuter, P.; Bockhorn, H.; Trimis, D.; (2019). Vortrag: Quasi-DNS of the Partially Premixed Sydney Flame. Simulation of reactive Thermo-Fluid Systems (STFS), Technical University Darmstadt, Germany , 22. September,
2018
-
Zhang, F.; Zirwes, T.; Nawroth, H.; Li, N.; Habisreuther, P.; Bockhorn, H.; Trimis, D.; Paschereit, C.O., (2018). Spectral Response of Different Combustion Models in LES of Direct Combustion Noise, in Proceedings of Joint Meeting of the German and Italian Sections of the Combustion Institute, Sorrento, Italy, 23-26 May, p. I8, (ISBN 978-88-88104-22-5), .
-
Zhang, F.; Zirwes, T.; Habisreuther, P.; Zarzalis, N.; Trimis, D.; Bockhorn, H., (2018). Large Eddy Simulation of Turbulent Flow in a Fan-Stirred Combustion Vessel, in Proceedings of Joint Meeting of the German and Italian Sections of the Combustion Institute, Sorrento, Italy, 23-26 May, p. I10, (ISBN 978-88-88104-22-5), .
-
Zhang, F.; Zirwes, T.; Habisreuther, P.; Bockhorn, H., (2018). Towards reduction of computational cost for large-scale combustion modelling with a multi-regional concept. Progress in Computational Fluid Dynamics, 18, (6), 333-346.(doi:10.1504/PCFD.2018.096616)
-
Zhang, F; Zirwes, T.; Habisreuther, P.; Bockhorn, H.; Trimis, D.; Nawroth, H; Paschereit, C.O., (2018). Impact of Combustion Modeling on the Spectral Response of Heat Release in LES. Combustion, Science and Technology, 191, (9), 1520-1540.(doi:10.1080/00102202.2018.1558218)
-
Zirwes, T.; Zhang, F.; Denev, J.A.; Habisreuther, P.; Bockhorn, H.; Automated Code Generation for Maximizing Performance of Detailed Chemistry Calculations in OpenFOAM. In High Performance Computing in Science and Engineering '17, Nagel W., Kröner D., Resch M. (ed.), Springer, Cham, p. 189-204, (doi:10.1007/978-3-319-68394-2_11) 2018.
-
Zirwes, T.; Zhang, F.; Habisreuther, P.; Denev, Jordan A.; Bockhorn, H.; Trimis, D.; Poster: Highly Resolved Numerical Simulation of Regime Transition in Mixed-Mode Flames. International Symposium on Combustion, Dublin, Ireland, July 29. - August 2., WIPP 1P116, 2018.
-
T. Zirwes; F. Zhang; J. A. Denev; P. Habisreuther; H. Bockhorn; D. Trimis; Poster: Generation of a Database with Detailed Numerical Simulation of Mixed-Mode Combustion. NIC Symposium 2018, February 22-23, ST 6, 2018.
-
Zirwes, T.; Zhang, F.; Habisreuther, P.; Denev, J.A.; Bockhorn, H.; Trimis, D.; Poster: A Reliability Assessment of Highly Resolved Numerical Simulation for Turbulent Combustion. Combustion-DNS Strategy & Data Analysis Workshop, Sorrento, Italy, 22-23 May, 2018.
-
Zirwes, T.; Zhang, F.; Habisreuther, P.; Denev, J.A.; Bockhorn, H.; Trimis, D.; Poster: Database and Prediction of the Regime Transition for the Sandia/Sydney Mixed-Mode Flame. Combustion-DNS Strategy & Data Analysis Workshop, Sorrento, Italy, 22-23 May, 2018.
-
Zirwes, Thorsten; Zhang, Feichi; Denev, Jordan A.; Habisreuther, Peter; Bockhorn, Henning; Trimis, Dimosthenis, (2018). Optimized Chemistry and Detailed Transport for Massively Parallel Simulations of Turbulent Combustion with OpenFOAM, in The 13th OpenFOAM Workshop (OFW13), vol. 13, June 24-29, Shanghai, China, .
-
Zirwes, T.; Zhang, F.; Habisreuther, P.; Denev, J.A.; Bockhorn, H., (2018). Automated Code Generation for Maximizing Performance of Detailed Chemistry Calculations in OpenFOAM. InSIDE, Innovatives Supercomputing in Deutschland, (Spring 2018),
-
Zirwes, T.; Zhang, F.; Habisreuther, P.; Denev, J. A.; Bockhorn, H.; Trimis, D., (2018). Optimizing Load Balancing of Reacting Flow Solvers in OpenFOAM for High Performance Computing, in Proc. of 6th ESI OpenFOAM User Conference, ESI-OpenCFD, Oct. 17-19, Hamburg, Germany , p. 1-13, .
-
Zirwes, T.; Zhang, F.; Denev, J. A.; Habisreuther, P.; Bockhorn, H.; Trimis, D., (2018). Detailed Transport and Performance Optimization for Massively Parallel Simulations of Turbulent Combustion with OpenFOAM, in The 13th OpenFOAM Workshop, 24.–29. June, Shanghai, China, p. 20-041 , .
2017
-
Feichi Zhang; Tobiast Baust; Thorsten Zirwes; Jordan Denev; Peter Habisreuther; Nikolaos Zarzalis; Henning Bockhorn, (2017). Impact of infinite thin flame approach on the evaluation of flame speed using spherically expanding flames. Energy Technology, 5, (7), 1055-1063.(doi:10.1002/ente.201600573)
-
Häber, T.; Zirwes, T.; Roth, D.; Zhang, F.; Bockhorn, H.; Maas, U.:, (2017). Numerical Simulation of the Ignition of Fuel/Air Gas Mixtures Around Small Hot Particles. Zeitschrift für Physikalische Chemie, (doi:10.1515/zpch-2016-0933)
-
Zhang, Feichi; Zirwes, Thorsten; Habisreuther, Peter; Bockhorn, Henning; A DNS Analysis of the Correlation of Heat Release Rate with Chemiluminescence Emissions in Turbulent Combustion. In High Performance Computing in Science and Engineering ´16, Nagel, Wolfgang E.; Kröner, Dietmar H.; Resch, Michael M. (ed.), Springer International Publishing, p. 229-243, (doi:10.1007/978-3-319-47066-5_16) 2017.
-
F. Zhang; T. Zirwes; P. Habisreuther; H. Bockhorn, (2017). Effect of unsteady stretching on the flame local dynamics. Combustion and Flame, 175, 170-179.(doi:10.1016/j.combustflame.2016.05.028)
-
F. Zhang; T. Zirwes; H. Nawroth; P. Habisreuther; H. Bockhorn; C.O. Paschereit, (2017). Combustion generated noise: an environment related issue for future combustion systems. Energy Technology, 5, (7), 1045-1054.(doi:10.1002/ente.201600526)
-
Zirwes, T.; Zhang, F.; Denev, J.; Habisreuther, P.; Bockhorn, H.; Zarzalis, N., (2017). Response of Local and Global Consumption Speed to Stretch in Laminar Steady-State Flames, in Proceedings of the European Combustion Meeting – 2017, April 18-21, Dubrovnik, Croatia, p. ECM2017.0379, .
-
Zirwes, T.; Zhang, F.; Häber, T.; Roth, D.; Bockhorn, H.:, (2017). Direct numerical simulation of ignition by hot moving particles, in International Colloquium on the Dynamics of Explosions and Reactive Systems, 26th , July 07 - August 04, Boston, USA, .
-
Zirwes, T.; Zhang, F.; Jordan A.D.; Habisreuther, P; Bockhorn, H.; Zarzalis, N, (2017). Effect of Elevated Pressure on the Flame Response To Stretch of Premixed Flames, in 28. Deutscher Flammentag, vol. VDI-Berichte 2302, VDI, 6.- 7. Sept., Darmstadt, Deutschland, p. 549-561, (ISBN 978-3-18-092302-4), .
-
Zirwes, T.; Zhang, F.; Denev, J. A.; Habisreuther, P.; Bockhorn, H.; (2017). Vortrag: Automated Code Generation for Maximizing Performance of Detailed Chemistry Calculations in OpenFOAM. 20th Results and Review Workshop of the HLRS, Stuttgart, Germany, Oct. 05-06,
2016
-
Feichi Zhang; Thorsten Zirwes; Peter Habisreuther; Henning Bockhorn; Holger Nawroth; Christian Oliver Paschereit; (2016). Vortrag: LES and DNS of Combustion and Combustion Generated Noise. 2nd Colloquium Combustion Dynamics and Noise, Villa Vigoni, Menaggio, Italy, Sept. 19-22,
-
F. Zhang; T. Zirwes; P. Habisreuther; H. Bockhorn; Numerical Simulation of Turbulent Combustion with a Multi-Regional Approach. In High Performance Computing in Science and Engineering ´15, Nagel, Wolfgang E.; Kröner, Dietmar H.; Resch, Michael M. (ed.), Springer International Publishing, Cham, p. 267–280, (doi:10.1007/978-3-319-24633-8_18) 2016.
-
F. Zhang; T. Zirwes; P. Habisreuther; H. Bockhorn; Poster: Identification of Correlation between OH* Chemiluminescence and Heat Release Rate with Direct Numerical Simulation. NIC Symposium 2016, 11-12 February 2016, Forschungszentrum Jülich, 2016.
-
Q. Zhao; F. Zhang; L. Zhang; H. Bockhorn; W. Xu; L. Liu, (2016). Multi-Regional Large Eddy Simulation of Turbulent Combustion. Journal of Propulsion Technology, 37, (2), 324 – 331.(doi:10.13675/j.cnki.tjjs.2016.02.017)
-
Zirwes, T.; Zhang, F.; Habisreuther, P.; Bockhorn, H.; (2016). Vortrag: A DNS Analysis of the Correlation of Heat Release Rate with Chemiluminescence Emissions in Turbulent Combustion. 19th Results and Review Workshop of the HLRS, Stuttgart, Deutschland, 13.–14. Oktober,
-
Zirwes, Thorsten; Zhang, Feichi; Habisreuther, Peter; Bockhorn, Henning; Poster: Flame Response to Unsteady Stretching. 36th International Symposium on Combustion, Seoul, Korea, July 31.-August 5., 36, 2016.
2015
-
F. Zhang; H. Bonart; T. Zirwes; P. Habisreuther; H. Bockhorn; N. Zarzalis; Direct Numerical Simulation of Chemically Reacting Flows with the Public Domain Code OpenFOAM. In High Performance Computing in Science and Engineering 14, Nagel, Wolfgang E. and Kröner, Dietmar H. and Resch, Michael M. (ed.), Springer International Publishing, p. 221-236, (doi:10.1007/978-3-319-10810-0_16) 2015.
-
F. Zhang; T. Zirwes; H. Nawroth; P. Habisreuther; C.O. Paschereit; H. Bockhorn, (2015). Direct combustion noise of premixed flames: experiments and simulation using compressible LES and DNS, in 15th International Conference on Numerical Combustion, Avignon, France, April, 19-22, .
2014
-
G. Geiser; A. Hosseinzadeh; H. Nawroth; F. Zhang; H. Bockhorn; P. Habisreuther; J. Janicka; C.O. Paschereit; W. Schroeder, (2014). Thermoacoustics of a turbulent premixed flame, in AIAA Paper, vol. 44, p. 2014-2476, (doi:10.2514/6.2014-2476).
-
F. Zhang; H. Bonart; P. Habisreuther; H. Bockhorn, (2014). Impact of Grid Refinement on Turbulent Combustion and Combustion Noise Modeling with Large Eddy Simulation, in High Performance Computing in Science and Engineering '13, vol. 16, Editors: Wolfgang E. Nagel, Dietmar H. Kröner, Michael M. Resch, p. 259 – 274, (doi:10.1007/978-3-319-02165-2_19).
-
F. Zhang; H. Bonart; P. Habisreuther; H. Bockhorn, (2014). Direct Numerical Simulation for an Assessment of the Correlation Between Heat Release Rate and Chemiluminescent Species in Turbulent Primixed Flames, in Joint meeting of the British and Scandinavian-Nordic Sections of the Combustion Institute, vol. 7, p. 23-24, .
-
Zhang, F.; Bonart, H.; Zirwes, T.; Habisreuther, P.; Bockhorn, H.; Poster: On Direct Numerical Simulation of Turbulent Combustion with OpenFOAM. NIC Symposium, Forschungszentrum Jülich, Germany, 12.–13. February, 2014.
2013
-
H. Nawroth; C. O. Paschereit; F. Zhang; P. Habisreuther; H. Bockhorn, (2013). Flow Investigation and Acoustic Measurements of an Unconfined Turbulent Premixed Jet Flame, in AIAA Paper, vol. 43, AIAA, p. 2013-2459, (doi:10.2514/6.2013-2459).
-
F. Zhang; H. Bonart; P. Habisreuther; H. Bockhorn, (2013). Direct Numerical Simulations of Turbulent Combustion with OpenFOAM, in Proceedings of the 26. Deutscher Flammentag, vol. 26, p. 867-872, .
-
F. Zhang; P. Habisreuther; H. Bockhorn, (2013). Application of the UTFC model to numerical simulation of turbulent combustion, in Chancen der Energiewende : wissenschaftliche Beiträge des KIT zur 1. Jahrestagung des KIT-Zentrums Energie, 19.06.2012. (KIT Scientific Reports ; 7640), vol. 1, p. 35-40, (ISBN 978-3-86644-985-5), (doi:http://dx.doi.org/10.5445/KSP/1000032863).
-
F. Zhang; P. Habisreuther; H. Bockhorn, (2013). Application of the unified turbulent flame-speed closure (UTFC) combustion model to numerical computation of turbulent gas flames, in High Performance Computing in Science and Engineering '12, Editors: Wolfgang E. Nagel, Dietmar H. Kröner, Michael M. Resch, p. 187-205, (ISBN 978-3-642-33374-3, online), (doi:10.1007/978-3-642-33374-3_16).
-
F. Zhang; H. Bonart; P. Habisreuther; H. Bockhorn; (2013). Vortrag: On Prediction of Combustion Generated Noise with help of Direct Numerical Simulation. Euromech Colloquium 546:Combustion Dynamics and Combustion Noise. Villa Vigoni, Menaggio, Italy, May 13-16, 2013,
-
F. Zhang; N. Sebbar; I. Auzmendi-Murua; P. Habisreuther; L. Zhang; H. Bockhorn; Large Eddy Simulation of DTBP Pool Fires. In Wissenschaftliche Beiträge des KIT zur 2. Jahrestagung des KIT-Zentrums Energie, Doktorandensymposium, Breh, Wolfgang; Sauer, Dominique (ed.), KIT Scientific Publishing, 13.06.2013, p. 103-108, (doi:10.5445/KSP/1000036425) 2013.
-
F. Zhang; P. Habisreuther; H. Bockhorn; H. Nawroth; C. O. Paschereit, (2013). On Prediction of Combustion Generated Noise with the Turbulent Heat Release Rate. ACTA ACUSTICA UNITED WITH ACUSTICA, 99, (6), 940-951.
2012
-
H. Nawroth; A. Saurabh; C. O. Paschereit; F. Zhang; P. Habisreuther; H. Bockhorn, (2012). Experimental and Numerical Investigation of a Turbulent Premixed Flame in an Anechoic Environment, in AIAA Paper, vol. 42, AIAA, p. 2012-3072, .
-
F. Zhang; P. Habisreuther; M. Hettel; H. Bockhorn, (2012). Numerical computation of combustion induced noise using compressible LES and hybrid CFD/CAA methods. Acta Acustica united with Acustica, 98, (1), 120-134.(doi:10.3813/AAA.918498)
-
F. Zhang; G. Geiser; P. Habisreuther; H. Bockhorn; W. Schröder; (2012). Vortrag: Impact of grid refinement on combustion noise prediction. Euromech Fluid Mechanics Conference - 9 (EFMC9), Rome, Italy, 9.-13.Sept.,
2011
-
F. Zhang; P. Habisreuther; M. Hettel; H. Bockhorn, (2011). Numerical simulation of noise emission from a non-premixed flame. Gaswärme International, 3/2011, 1-6.
-
F. Zhang; P. Habisreuther; M. Hettel; H. Bockhorn, (2011). A newly developed unified TFC combustion model for numerical simulation of turbulent gas flames, 25. Deutscher Flammentag, Karlsruhe, 14. und 15. September 2011, p. 177-182, .
2010
-
F. Zhang; P. Habisreuther; M. Hettel; H. Bockhorn, (2010). Application of a Unified TFC Model to Numerical Simulation of a Turbulent Non-Premixed Flame, in Proceedings of the 8th International ERCOFTAC Symposium on Engineering Turbulence Modelling and Measurements - ETMM8, vol. 2, European Research Collaboration on Flow Turbulence and Combustion, ERCOFTAC, June, 9-11, Marseilles, France, p. 681-686, .
-
F. Zhang; P. Habisreuther; M. Hettel; H. Bockhorn; (2010). Vortrag: Application of a unified TFC model to numerical simulation of a lifted turbulent partially premixed flame. Euromech Fluid Mechanics Conference - 8, Bad Reichenhall, Germany, 13-16. Sept.,
2009
-
C. Bender; F. Zhang; P. Habisreuther; H. Büchner; H. Bockhorn; Measurement and Simulation of Combustion Noise emitted from Swirl Burners. In Combustion Noise (DFG Research Unit 486), Anna Schwarz, Johannes Janicka (ed.), Fluid Mechanics and its Applications, Springer, Berlin Heidelberg, p. 33-62, (doi:10.1007/978-3-642-02038-4_2) 2009.
-
F. Zhang; P. Habisreuther; M. Hettel; H. Bockhorn; (2009). Vortrag: LES of a Complex Premixed Swirl Burner. Colloquium of the European Mechanics society on Large Eddy Simulation for Aerodynamics and Aeroacoustics, TU Müchen, 23-25, March,
-
F. Zhang; P. Habisreuther; M. Hettel; H. Bockhorn, (2009). Numerical Investigations of the Noise Sources Generated in a Swirl Stabilized Flame. Acta Acustica united with Acustica, 95, (3), 418-427.(doi:10.3813/AAA.918166)
-
F. Zhang; P. Habisreuther; M. Hettel; H. Bockhorn, (2009). Modelling of a Premixed Swirl-stabilized Flame Using a Turbulent Flame Speed Closure Model in LES. Flow, Turbulence and Combustion, 82, (4), 537-551.(doi:10.1007/s10494-008-9175-x)
2008
-
F. Zhang; P. Habisreuther; M. Hettel; H. Bockhorn, (2008). Numerical investigations of the noise sources generated in a swirl stabilized flame, in Fortschritte der Akustik - DAGA 08, 34. Jahrestagung für Akustik, DAGA '08, .
-
F. Zhang; P. Habisreuther; H. Bockhorn, (2008). LES of reactive flow in a strongly swirling combustor system, in International Conference on Jets, Wakes and Separated Flows, Berlin, .
2007
-
P. Habisreuther; F. Zhang; H. Bockhorn; (2007). Vortrag: Investigations on the noise emissions of a premixed swirl burner using LES. 11th CEAS-ASC Workshop, Lissabon, Portugal, 27-28.Sept.,
-
F. Zhang; P. Habisreuther; M. Hettel; H. Bockhorn, (2007). Modelling of a Premixed Swirl-Stabilized Combustor using a Turbulent Flame Speed Closure Model in LES, in Computational Combustion 2007, Proc. 2nd ECCOMAS Thematic Conference, ECCOMAS, Delft, Netherland, 18./20. Juli, .
-
F. Zhang; P. Habisreuther; M. Hettel; H. Bockhorn, (2007). Computational Method on Prediction of Combustion Noise, in Proc. of the 19th International Congress on Acoustics, International Commission for Acoustics, ICA, Madrid, Spain, 2.-7. September, .